
THE ENERGY CHALLENGE 2004 -- Wind
10.13.04
Murray Duffin, Retired
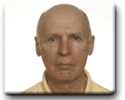
In addressing the declining availability of fossil
fuels, and with nuclear energy less than popular,
the remaining choices are energy efficiency and renewables.
Fortunately, they are complementary choices and have
the added virtue of being carbon free. Renewables
include hydro, wind, solar, bio-fuels, geo-thermal,
wave, and tidal energy. Of these, wind, solar, geo-thermal,
and wave/tidal are abundant, but only wind is currently
economical and easy to harness.
Whatever you think you know about wind, especially
the negatives, if it was based on data and analysis
prior to 2000 you can be pretty sure it's wrong. Wind
has made enormous strides in the last 15 years. In
1992 the average size of installed wind turbine was
200 kW. In 2002 it was 1.4MW. Now almost all units
being installed are over 2 MW. Total world installed
wind energy in 1992 was 2.5 GW. By 2003 it had reached
40 GW, a CAGR of 30%/yr.
How Much Energy
Probably the best early data on the total USA wind
resource is the 1993 report found at www.nrel.gov/wind/potential.html.
This report estimated total potential for 25% efficient
turbines, with 25% losses, and average 50m hub heights,
and made exclusions for environmental, urban, and
agricultural purposes. The result was that about 15
quads of equivalent fossil fuel energy could be replaced
by class 5 to 7 winds. Adding class 4 winds, which
were marginal at that time, raised the potential to
greater than 60 quads. Most recent Texas wind farms
are in class 4 areas.
This report was based on 1991/92 technology, when
the largest envisioned turbines were 300 KW and blade
rotation speeds were such that considerable areas
were excluded for environmental reasons, i.e. bird
kill. Best wind speeds were 15-25 mph and it was also
assumed that only 20% of the actual wind energy/km2
could be converted to electricity.
Now for 1.5 MW turbines with hub heights near 80
meters, Archer and Jacobson1 find class
3 winds are economic, and are available for about
20% of the lower 48 land area. They also have found
that near shore coastal areas with suitable winds
cover more than 2x the shoreline of the 1993 paper.
Today turbines being installed are up to 3 MW and
up to 5 MW are in development. Blade rotation is much
slower. Efficiencies are now above 30 % and losses
below 15%. Productive wind speeds are now in the class
3 range. Conservatively, total lower 48 available
wind energy with 2004 technology is in the order of
150 quads, fossil fuel equivalent, or 50% more than
total USA current primary energy demand. We are unlikely
to want to harness more than 1/4th of that between
now and 2050.
Intermittency
The primary problem usually raised by wind opponents
is intermittent availability with significant daily,
monthly, and seasonal variations. Probably the first
person to address this issue systematically was Gregor
Czisch for Western Europe. He analyzed 3 hr. interval
recorded wind speed (at 10 m average height above
ground) for all areas that could provide =>1500
full load hours (FLH)/yr, i.e. minimum 17% full load
factor. His analysis shows that:
- 5 minute correlation is near zero at 20 km
- 12 hour correlation is near zero at < 1000
km
- 24 hour correlation is near zero at 1800 km
- monthly correlation is near zero at 2500 km.
Of course at the 80 m hub height of a 1.5 MW turbine
the FLH and correlation distances would improve significantly.
Archer and Jacobson (A&J)1,2 found
that for a small area only 500 by 700 km centered
in Kansas, averaged over 8 wind-farm locations, the
incidence of zero power wind was zero.
One turbine might be expected to produce 30% of
rated kWh during a year. Using the 8 wind farm curve
of average windspeed vs % of time available, and assuming
the ratings of the NEG/Micon NM82/150 turbine (nominal
windspeed of 12 m/s, cut in windspeed of 3 m/s and
cut out windspeed of 18 m/s) the 8 wind-farms produce
85.5% of nominal annual output and operate at or above
nominal 38% of the time.
However this estimate understates probable performance
for 3 reasons:
- A&J used measured wind speed increase from
10 m to 80 m on a few sites, generated a formula
to be applied to all other sites where measurements
at 80 m were not available, and generated their
curve using the estimated 80 m windspeed. Because
wind speed increase is not linear with height, and
because power is proportional to the cube of windspeed,
the upper half of the swept circle has more weight
than the lower half. The “virtual” windspeed
at the hub is higher than the estimated.
- Turbine manufacturers specify performance parameters
conservatively.
- Measured upper level wind speeds tend to be slightly
higher then estimated.
Therefore, as a conservative adjustment, to better
reflect expected performance, the A&J 8 wind-farm
curve was shifted right by 1 m/s and performance recalculated.
With this adjustment, for the selected turbines, the
8 wind-farms can be expected to produce 111% of nominal
energy in a year, and would be at =>100% of nominal
output 48% of the time. With wind turbine costs now
at about $0.90/W installed, and amortization over
30 years at 6 % the direct cost of electricity at
nominal output would be 1.91 cents/kWh. If we increased
the number of turbines by 33% the cost of electricity
at nominal output would go to 2.54 cents/kWh, we would
be at =>nominal output 58% of the time and we would
generate 147% of nominal output energy per year.
If we added hydrogen fueled gas turbine backup at
40% of nominal power at a capital cost of $.60/W financed
at 6 %for 30 years we would be at => nominal output
75% of the time and nominal electricity would go up
to 3.14 cents/kWh. Total output would go to 157% of
nominal. If the surplus energy is used to generate
and store hydrogen at 75% efficiency (feasible with
existing electrolysis and compression equipment),
and the backup burns hydrogen to generate electricity
at only 40% efficiency (greater than 50% should be
possible with a CCGT), there would be at least 70%
more hydrogen than needed to run the backup generator.
The cost of the hydrolysis, compression and storage
might push the direct cost for total nominal electricity
to 3.5 cents/kWh. This cost is better than coal or
natural gas at 2004 prices.
Now extend this approach to even more efficient
3 MW turbines and perhaps 3 times as many wind-farms
spread over say 500 by 2000 km. and nominal power
will be available close to 100% of the time, so the
problem of intermittence can readily be overcome.
However, to get there utility management would have
to think in whole system terms and would have to cooperate
over a large interstate geographic area, a couple
of things they are not accustomed to doing.
A Possible Surprise
If it will scale up an even more exciting potential
has been illustrated by a 9th grade Canadian girl.6
A dual rotor turbine has the potential to harness
lighter winds, lowering cut-in and cut-out speeds,
and greatly increasing the harnessable wind resource.
Alternatively the 2 rotor approach could enable significantly
smaller rotors for the same wind regime. The 2 rotor
approach might also lower turbine cost by enabling
a more balanced design. There is some possibility
that 3 rotors would provide additional improvements,
but perhaps not enough to be cost effective.
Certainly this possibility calls for immediate analysis
by the wind industry, even if the source might prove
to be a bit embarrassing.
Operation
To make such a system work effectively we need three
additional elements, good hourly to daily wind forecasting,
computerized dispatching and load matching, and a
well-integrated transmission network. The keys to
smooth operation that have been listed by various
experts are:
- variable speed and power factor turbines
- modern control systems with remote sensing and
control
- regional wind forecasting up to 2 days ahead
- local wind forecasting up to 2 hours ahead
- minimum start and stop transients
- good smoothing of individual turbine outputs
in wind-farm outputs
- wind-farm policy integrated into regional utility
policy.
All of these are common sense, manageable requirements.
Wind antagonists raise cost issues of connections
to the grid, and the costs of ancillary services due
to wind variability. In many cases the output from
wind-farms can serve local communities, thus reducing
load on regional grids. However large scale development
of wind power will require upgrades of regional and
national grids. Any energy policy must strongly address
upgrading and development of the transmission infrastructure.
Wind should be central to such planning and execution.
This is simply not a wind specific issue. Several
studies3 have been done to cost the ancillary
services with resulting estimates from 0.2 to 0.6
cents/kWh with wind from 5 % to 20% of the local total
energy supply. The worst case includes day ahead forecast
errors of 50%. With a well integrated network of wind-farms
as described in 3) above, these already very small
costs would decline
Cost
In a 1995 disinformation effort, the coal industry
sponsored a report developed by Resource Data International
and published by the Center for Energy and Economic
Development, projecting wind energy costs of 6.8¢/kWh
in 1995, remaining unchanged until 2010.1
In a rebuttal, NREL estimated 5.3¢/kWh in 1995, going
to 3.5¢ in 2010.1 The Lake Benton Wind
Farm in Minnesota, now in production, produces electricity
at 4 cents/kWh unsubsidized, using 1 MW turbines.
With larger turbines the cost would be lower. Of course
the cost will vary with wind class and siting issues,
but for developments we are likely to see by 2010,
the NREL estimate is looking good. We can expect average
costs in the future to be cheaper than coal fired
plants, with none of coal’s environmental issues.
Objections5
The usual objections presented by wind skeptics
are:
- Bird kill
- Unsightliness
- Land area
- Noise
- Low energy returned on energy invested
- Future like the past
In response to these objections one can state:
Bird kill – The only place that has posed
a real problem was the Altamont pass in the 1980s,
with small fast rotating turbines. There is no evidence
that new large turbines, with slowly rotating blades,
kill even as many birds as power lines do4.
Unsightliness – Surveys in Palm Springs and
Wales (UK) show that neighbors grow to like wind farms
and find them attractive. Most wind farms in the USA
will be sighted in areas that vary from rural to empty,
where the issue is unlikely to arise.
Land area – Class 4 and higher wind areas
available for wind development are 6% of total lower
48 land area. Of this area, less than 5% would be
occupied by turbines, equipment, and access roads.
Cultivation can be carried out almost to the base
of the turbines, and livestock like the wind shadow.
Noise – Modern turbines have noise levels
below 50 dbm (like a summer breeze in the trees) at
distances of about 250 yards.
Low EROEI - A recent study at the University of
Wisconsin-Madison finds that wind farms generate between
17 and 39 times as much energy as is required for
their construction and operation. The Danish wind
energy association comes up with an energy payback
time of less than 6 months, or a return of >60
for a 30 year life.
Future like past – Saying that wind will never
happen, because it never has is like saying a one-year-old
will never walk because he never has.
Benefits
Perhaps the major benefits are environmental. There
is one well documented and quantified example to support
this advantage: In 2001, Ontario Canada’s five
coal fired power plants were responsible for 20% of
all greenhouse gases released in the province, 23%
of all sulphur dioxide emissions, 14% of nitrogen
emissions and 23% of mercury emissions. These plants
are scheduled for closure by 2007.
More specifically, one can say for wind that:
- It’s not a source of nuclear waste.
- It’s does not despoil the land like strip
mining for coal.
- It does not damage fragile habitats, like drilling
for coal bed methane.
- It does not threaten the ANWR.
- It’s not a source global warming greenhouse
gases.
- It’s not a source of fish-contaminating
mercury.
- It’s not a source of acid rain.
Apart from clean, inexpensive power, the surprise
benefits to the economy can be a drop in farm subsidies.
Minnesota farmers earn less than $30/acre with livestock,
and $250 per acre with crops, but can earn $1,000/acre
from land rental for wind farms, and still have the
livestock or crop.
The big benefit to operators is freedom from fuel
price risk, and that benefit will only grow from an
already very attractive level in 2004.
The Challenge
Several states have goal of getting 10 5 of their
electricity from wind by 2015 or 205 by 2020. With
declining availability of natural gas and oil, we
will have to do much better than that on a national
basis. The real goal should be to get perhaps 20%
of our total energy (albeit a declining total) by
2030 or 2040.
A 2 MW wind turbine with a 30% duty cycle and 95%
availability will generate 5.8 million kWh/year. Fifteen
quads of wind power by 2030 would require 750,000
turbines, or 30,000 per year starting now. That is
five times present world production capacity, but
is probably a worst-case estimate. At 3MW, 35% duty
cycle and 15 quads we would need only 450,000. Building
15,000 to 30,000 turbines per year is no big deal
for an economy that can build 17 million cars, trucks,
and busses per year, but still, we had better get
cranking. It can’t wait until after 2020.
Could the 2 rotor design mentioned above reduce
dramatically the number of installations needed? The
wind industry needs to address this question urgently.
References:
1 http://www.stanford.edu/group/efmh/winds/winds_jgr.pdf
2 http://fluid.stanford.edu/~lozej/winds/winds.html
3 http://www.nrel.gov/wind/pdfs/grid_integration_studies_draft.pdf
4 http://www.awea.org/faq/sagrillo/swbirds.html
5 http://www.eere.energy.gov/windpoweringamerica/
6 http://www.alumni.ca/~walk4d0/sf11.html
Readers Comments
Date |
Comment |
George Fleming
10.12.04 |
Glad to see such a favorable article on wind
power. Fine arguments and documentation. A Korean
company called Wintec built some wind turbines
with two rotors, a larger one upstream and a
smaller one downstream. Prototypes were about
0.5 MW as I recall. However, I am not sure this
company is still in business. I wasn't able
to access their website today, www.wintec4nature.com,
but printed several pages from it earlier this
year.
|
Steve Sturgill
10.13.04 |
Murray, I need some help locating the UW EROEI
study you mentioned. I very much want to believe
that wind energy's infrastructural EROEI is
high, but I need to see how the conclusion was
reached. Please post a link or some additional
information so that I can find the study. Thanks.
|
Tom Gray
10.13.04 |
Steve,
I think http://fti.neep.wisc.edu/FTI/pdf/fdm1063.pdf
is probably what you are looking for.
Tom Gray American Wind Energy Association
|
Len Gould
10.13.04 |
Murray: A commendable article, and I must say
somewhat reluctantly that I essentially agree
with all your points except the feasibility
of young Dayna Walker's proposal for multiple
turbines. If you notice, she's not proposing
counter-rotating turbines, but co-rotating turbines.
It has long been well known, as even her own
discussion points out, that increasing the blade
coverage percent can increase power out, but
it also severely restricts the upper range of
wind speed which can be used before needing
to shut down, witness the old prairie water
pumps. Much science has already gone into sorting
out the optimal figures for this and I don't
see that her efforts, commendable as they are
for her age, contribute anything new. However,
if you suggested multiple co-rotating turbines
where individual blade groups could be feathered
and halted as the wind speed increased, you
might have something, though there would definitely
be turbulence problems as the remaining working
blades passed the halted blades. Probably not
worth it.
I'd always thought that wind generation was
limited in reliability to a maximum of about
30% with many real-life installations not doing
better than half that, but I've just come across
a new contract just issued by Hydro Quebec for
new wind farms (GE) on Canada's east coast.
They've signed a solid contract with two developers
for a total of 990 mW of wind turbines which
will, by contract terms, generate 3,200 twhr
per year of energy. That means they're going
to generate at 36.87% on an annual basis including
breakdowns, maintenance, and etc. It occurs
to me that these guys have taken advantage of
one of the complexities of wind power, which
is that "it doesn't matter for reliability how
fast the average wind speed is, what matters
is how steady it is." !! Neat. If the wind speed
is low but continuous, they simply need to install
larger rotors, which costs very little extra.
Find a location with steady, even, continuous
wind speeds and you can do much better than
even the places with the highest peak speeds
and total power. The suppliers to Hydro Quebec
state they can profitably sell wind power to
the utility for Cdn$0.065 / kwhr with NO SUBSIDIES
using GE turbines at an average cost of Cdn$1,800
/ kw. Ov course hydro Quebec has the huge advantage
of their enormous hydro generation facilities
which can happily store the water whenever the
wind is blowing, while releasing it for generation
whenever needed. Looks like they're making an
effort to become North America's greenpower
supplier.
|
Len Gould
10.13.04 |
Steve: Try also the ExternE study at http://externe.jrc.es/infosys.html
or the WNA at http://www.greatchange.org/bb-thermochemical-WNA_energy_analysis_of_power_systems.html
. Eg at the WNA site the differences between
diffusion fuel processing and centrifuge processing
are laid out for you. (The fact that the Wisconsin
study cited by Tom used only the obsolete diffusion
process figures makes me suspicious of their
hidden agenda) Overall, nuclear's input/output
ratio is closer to double the number stated
in the Wisconsin so-called "study".
|
Peter Bradford
10.15.04 |
An interesting
and thorough article. Thank you.
However, your discussion of unsightliness
did not mention a controversy that goes with
the newer and larger towers, namely that they
must be so tall that they are required to be
topped by bright lights (in the U.S. at least)
to warn off airplanes. This makes them especially
controversial in mountainous areas with scenic
ridgelines and tranquil night skies.
Wind turbine sighting prospects in such areas
would be much improved if the wind industry
would work with the Federal Aviation Administration
to develop alternative warning systems. After
all, the ridges are often in clouds anyway,
so the lights can't be the sole warning system.
A warning system that worked by sound or that
turned on its lights only when a plane was in
the area would reduce the intensity of sighting
controversies substantially.
|
George Fleming
10.17.04 |
Mr. Gould's comment
about blade coverage sent me looking for more
information. The Wind Turbine Co. (www.windturbinecompany.com/technology/index.html),
which has been developing two-blade downwind turbines,
says: "It is well known that 2-blade turbines
capture approximately 97% as much energy as 3-blade
machines with the same rotor diameter." Several
single-blade turbines have been built and tested,
according to the book "Wind Power Plants" (Gasch
and Twele: Solarpraxis AG, 2002). One of these
was called MONOPTEROS (640 kW), and it looks something
like a monster. These single-blade turbines worked,
but the book does not say why they did not succeed
in the market. I would like to see further comments
on this subject by Mr. Gould and others.
|
Murray Duffin
10.17.04 |
Len, - Clearly
I am not an expert on wind turbines, but I have
searched for info. on multi rotor experience and
find a paucity of information. What struck me
about Dayna Walkers results was the sheer magnitude
of the improvement. Also my experience tells me
that experts often end up unable to think outside
the box. It may be that Dayna's work represents
nothing new and her results are just an artifact
of poor or limited experimental design. I hope
not. Intuitively, it seems to me that her approach
can lower the cut-in wind speed, increase the
low wind speed output, and that feathering of
the downwind rotor could raise the cut-out wind
speed. The result could be to increase the percent
of time that nominal power was reached quite considerably,
thus further mitigating the intermittancy problem.
There was a brief note in the Scientific American
a couple of months ago about an experimenter who
wondered why some whales have bumps on the leading
edge of their flippers. He wind tunnel tested
wing sections with and without bumps and found
that the bumps increased lift, reduced drag, and
lowered stall speed. This seems like another outside
the box idea for wind turbine design. Peter, there
are always peripheral issues to address, like
tower lights. Most wind farms will not be located
in scenic mountain areas, and if the choice is
energy scarcity or lights we will take lights,
or as you suggest find other solutions. Most of
the negatives that have been raised about wind
are much more serious and it was those issues
that I wanted to address. Murray
|
Len Gould
10.17.04 |
Murray: I agree,
many experts often lock themselves into boxes
with what they know and it often takes a non-expert
to break out. Check Doug Selsam's multi-rotor
designs at http://www.speakerfactory.net/wind.htm
. This concept is particularly an interesting
out-of-box development. The system eliminates
costly controls by simply installing the long
shaft on a spring-loaded tilt platform. In low
wind each rotor sees full wind. At higher wind
speeds wind pressure pushes the rearward rotors
down until they are shadowed by the ones in front.
I've been following this for a few years and he's
making excellent progress in development. Should
be cheap to mass produce.
A good discussion of blade fill percentage
and count is at the Danish Wind Industry Org.
site at http://www.windpower.org/en/tour/design/concepts.htm
" Two- and one-bladed machines require a more
complex design with a hinged (teetering hub)
rotor as shown in the picture, i.e. the rotor
has to be able to tilt in order to avoid too
heavy shocks to the turbine when a rotor blades
passes the tower. The rotor is therefore fitted
onto a shaft which is perpendicular to the main
shaft, and which rotates along with the main
shaft"
also at http://www.windpower.org/en/tour/design/optim.htm
"The water pumping windmills to the left look
very different from modern, large wind turbines.
But they are quite sensibly designed for the
purpose they serve: The very solid rotor with
many blades means that they will be running
even at very low wind speeds, and thus pumping
a fair amount of water all year round. Clearly,
they will be very inefficient at high wind speeds,
and they will have to shut themselves down,
and yaw out of the wind in order to avoid damage
to the turbine, due to the very solid rotor.
"
|
Len Gould
10.17.04 |
Further
Wind turbines are built to catch the wind's kinetic
(motion) energy. You may therefore wonder why
modern wind turbines are not built with a lot
of rotor blades, like the old "American" windmills
you have seen in the Western movies. Turbines
with many blades or very wide blades, i.e. turbines
with a very solid rotor, however, will be subject
to very large forces, when the wind blows at a
hurricane speed. (Remember, that the energy content
of the wind varies with the third power (the cube)
of the wind speed). Wind turbine manufacturers
have to certify that their turbines are built,
so that they can withstand extreme winds which
occur, say, during 10 minutes once every 50 years.
To limit the influence of the extreme winds turbine
manufacturers therefore generally prefer to build
turbines with a few, long, narrow blades.
see http://www.windpower.org/en/tour/design/index.htm
One, two or three blades can capture as much
power from a given wind as much more solid rotors
simply by turning faster. Also, you can't get
any energy from a wind if you slow it down too
much. You wind up with static air behind your
turbine and the wind just going around the whole
thing. I've seen the math for this concept in
a few places but can't find a reference right
now.
|
Scott White
10.18.04 |
In response to
Len Gould's comments about my study at the University
of Wisconsin: there was no hidden agenda. When
analyzing uranium enrichment, the diffusion process
was used only because it was the sole enrichment
process used in the U.S. at the time. While enrichment
using gas centrifuges or lasers are considerably
more energy efficient than gaseous diffusion,
neither were being used in as of 1998, when the
study was completed. At that time there were two
gaseous diffusion enrichment plants in operation
- one at Portsmouth, Ohio, the other at Paducah,
Kentucky. As I understand it, only the Paducah
plant is still operating. When enrichment plants
using the gas centrifuge or lasers become built
in the U.S., then this study should be updated.
As for the study you reference in the same
response, I have yet to study it to see why
it's results differ from my own.
Also, relating to wind energy, the study results
of wind are currently being reexamined and I
plan to present the updated results at the 2005
American Wind Energy Association conference
in Denver next May. At the time the original
study was published, 2 of the 3 windfarms analyzed
did not have a full years' worth of production
data to figure into the analysis, which meant
the production data was based on the operators
projections. Since there are now at least 6
years of generation data to base these projections,
the results have changed somewhat. For more
information on the wind results, see the open-file
report for the wind data at: http://fti.neep.wisc.edu/FTI/pdf/fdm1092.pdf,
which has more details then the refereed article
Tom Gray pointed out.
|
Len Gould
10.18.04 |
Scott: Thanks
for the new data. I didn't realize that the centrifuge
system wasn't yet being use in the US. Perhaps
to present a fair picture of the potential of
nuclear power it might have been prudent to reference
other countries systems
"Some reactors, for example the Canadian-designed
Candu and the British Magnox reactors, use natural
uranium as their fuel. "
"A number of enrichment processes have been
demonstrated in the laboratory but only two,
the gaseous diffusion process and the centrifuge
process, are operating on a commercial scale."
Surely this last bit of data wasn't unavailable
to you only 5 years ago.
"The gaseous diffusion process consumes about
2500 kWh (9000 MJ) per SWU, while modern gas
centrifuge plants require only about 50 kWh
(180 MJ) per SWU."
|
Graham Cowan
10.18.04 |
Actually I see
White
and Kulcinski's paper says the basis
of its uranium enrichment energy estimates was
in fact gas centrifuge enrichment. In its table
5 it shows, for fuel preparation, 1203 thermal
TJ per electrical gigawatt-year. Supposing 33
percent heat-to-electricity conversion that's
1.26 percent of the yield of the fuel produced,
which is indeed less than the roughly two percent
that gaseous diffusion nowadays takes.
It's not as much less as I would have expected
given the 50-fold reduction, mentioned here,
in enrichment's electricity needs when centrifuges
replace gaseous diffusion, but there are other
parts to the fuel-preparation process besides
enrichment, I suppose.
Windpower is favored in that paper, as it acknowledges,
by its ignoring of the energy cost of storage,
or as in current systems, of calling in other
kinds of power plant that can pick up when the
wind weakens.
If that would knock wind's lifetime EROEI from
27 down to 20, still beating the figure there
computed for nuclear, that, in my opinion, would
be just another illustration of the unimportance
of EROEI (as long as it's above ~2).
This emerged in another discussion where solar-concentrating
heat engines looked as if what I suppose is
the dominant EI in making them, the mirrors,
turned out to take only a few weeks' worth of
their output to make. Labor was the scarce good
they really wasted. Or, as a certain kind of
advocate would say, job creation was one of
their major benefits.
--- former hydrogen fan Graham Cowan
how
personal mobility gains nuclear cachet
|
Scott White
10.18.04 |
Graham is correct in that our study did use
gas centrifuge and not gaseous diffusion - I
should have actually gone back and looked at
it before responding earlier. My apologies.
And to Len, yes we did know about the gas centrifuge
and did opt to use that data instead of diffusion.
I'm still not sure why the discrepancy is so
large, but will not be able to revisit this
until later in the week due to some other business.
And as Graham did note, we did clearly state
that the wind energy results benefit from not
considering energy storage. That is for another
study. To compare apples to apples (and baseload
to baseload) energy storage should be factored
in when comparing intermittent technologies
to baseload ones. However, in small enough doses,
there is enough load-following capacity to both
allow intermittent technologies (such as wind)
onto the grid and to justify studying them as
stand alone as we did.
Denholm and Kulcinski did a study on energy
storage in 2003. The results here showed that
the EPR of wind dropped from 23 to 17 using
pumped hydro storage and to 10 with compressed
air storage. This report can be viewed at: http://www.ecw.org/prod/223-1.pdf
|
|