
Distributed Energy Resources: Why IEEE 1547 won't
be the Last Word
3.2.05
Roger Arnold, Owner, Silverthorn Engineering
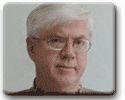
DG: Is it time yet?
Advocacy for distributed generation (DG) has a long
history. Conservation activists preached the concept
in the early '70s, and even before. But it never really
caught on. Now, suddenly, it's again become a hot
topic. Grid-connected PV systems are blossoming on
individual homes, and Capstone Microturbines is having
some success in selling small systems for combined
heat and power (CHP) at commercial and small industrial
sites. A United States Combined Heat and Power Association
is raising its voice in Washington, and the Department
of Energy has major programs devoted to distributed
energy resources (DER).
So what has happened, and what does it mean for
electrical power systems in the future?
Not everyone would agree that anything of real significance
actually has happened. Overall, the DG market remains
quite small, accounting for something on the order
of 1% of total US electricity supply. The bulk of
that is from a comparatively small number of large
industrial CHP applications, many of which have been
in place for decades. DG skeptics don't see much reason
for the situation to change significantly.
Nevertheless, a number of things have changed to
boost the potential for DG and DER.
What's different?
As often seems to be the case when markets turn
a corner, it's not really one big thing that does
the trick, but an accumulation of smaller things.
Many individual developments have come together to
tip the economic scales on DG from mostly unfavorable
to increasingly favorable.
Perhaps the most significant development is simply
the ongoing cost-performance improvement in power
electronics and controls. That has made it feasible
to develop smart inverters that are efficient, economical,
and safe for connection to the power grid. The new
IEEE 1547 "Standard for Interconnecting Distributed
Resources with Electric Power Systems" has facilitated
the process by giving equipment designers a single
well-regarded standard to work to. Underwriter Laboratories
standard UL 1741 references IEEE 1547 for its technical
requirements. The UL standard is now the basis for
certification of DG interconnection equipment. Although
the utility industry is highly fragmented, UL certification
carries weight. Together, IEEE 1547 and UL 1741 bring
a degree of confidence and regularity for the DG equipment
industry that was sorely lacking in the past.
Another change has been the shifting economics of
various power resources. The price of gas and coal,
the chief fuels for central power generation, have
risen sharply in the last few years, while the economics
of wind and solar power generation have been steadily
improving. Also, the recent development of reliable,
low cost micro-turbine systems has brought the efficiency
of CHP systems within reach of smaller businesses
for whom it wouldn't previously have been a viable
option. The turbines, which can run on very lean gas
mixtures, have also made it feasible for cities to
tap gas from landfills and sewage treatment plants
as DG resources.
At least as significant as the changes that have
already occurred are those that are expected in the
near future. Chief among those is the economic feasibility
of fuel cells for stationary power generation. These
will be high temperature units that can potentially
be fueled by hydrogen, internally reformed natural
gas, or synthesis gas from coal or biomass. High temperature
operation will enable them to be coupled with microturbines
for very efficient power generation. Several competing
technologies are already established well enough for
test deployment. They are currently too expensive
to threaten micro-turbines in CHP applications, but
with the amount of development attention they are
getting, that will almost certainly change. They promise
to double the thermal efficiency of power generation
compared to present micro-turbines, and rewrite the
economic equations for CHP.
And then there's wind power:
Wind power is perhaps the strongest driver for DG
technologies. In good locationsand disregarding
the cost of backup power and new transmission lineswind
turbines can now deliver energy at a lower cost per
kWh than either gas or coal fired plants, and with
zero CO2 emissions. Transmission issues are a big
concern, as is intermittent supply, but wind power
is nonetheless an increasingly attractive option on
an economic basis alone. If CO2 emissions are ever
taxed, its advantages will be impossible to ignore.
Wind power is an odd duck in the usual scheme of
central vs. distributed power. Wind turbines are necessarily
spread out geographically. Individually, they produce
power as distributed resources, yet they don't look
much like any other DG resource. The output from dozens
to hundreds of turbines is aggregated in wind farms
whose net capacity may compare to that of central
power plants. Wind farms tend to lack the signature
DG advantage of low transmission costs by virtue of
being physically close to where most of their output
is consumed. On the contrary, their irregular output
and distance from markets can be a major challenge
for power transmission systems.
Ironically, the most cost-effective strategy for
utilities to integrate high levels of wind power may
be to take advantage of other distributed energy resources.
However, that will need to include discretionary (dispatchable)
loads as well as distributed generators. It will also
require a more advanced standard for interconnecting
these resources than what is provided by IEEE 1547.
To see that, let’s first take a closer look
at what’s involved in 1547.
Anti-Islanding
The key requirement that shaped IEEE 1547 is reliable
anti-islanding. "Islanding" refers to the
creation of an "island" of energized lines
drawing from a local generator running independently
of the grid at large. It can be deliberate, as when
an industrial facility with its own generating capacity
isolates itself from a stressed grid, in order to
avoid voltage sags and risk of blackout. But it can
also occur unintentionally, if a DG resource fails
to isolate itself when the grid to which it is connected
goes down. An island of energized lines persists in
the middle of a region of what are supposed to be
dead lines. That can be a life-threatening hazard
for line crews working in the area.
The approach specified in 1547 for anti-islanding
involves a combination of voltage and frequency feedback.
Basically, the inverter will only output power to
the line if it detects AC voltage on the line indicating
an active connection to a central generator. If the
voltage goes either too high or too low, the inverter
immediately disconnects.
To make sure that the voltage it is sensing is truly
that from a central generator, and not the output
of another DG resource or the echo of its own output
from a reactive load, all 1547-compliant devices share
a certain design feature. They "try" to
output power at a frequency slightly higher than the
standard line frequency. But they also use phase-locked
loop methods to continuously sync their output with
that of the power line. As a result, they never actually
go out of phase with the central generator, as long
as the connection holds. If the connection is lost,
however, and a coincidental match between active DG
resources and the set of loads prevents the voltage
from immediately dropping out of range, the set of
DG resources driving the line will find themselves
"freewheeling" at their set frequency somewhat
above line frequency. An internal clock quickly detects
the resulting phase drift, and shuts down the output.
This approach has the advantage that it is relatively
simple and depends only on the line connection. It
does not require any side channel communication, and
it can be implemented in a certifiable fail-safe manner.
But it also has intrinsic limitations that will eventually
necessitate something more advanced.
Limitations of 1547
The most obvious limitation of IEEE 1547 is that,
by design, it requires a strong central generator
to provide the "heartbeat" for the system.
DG resources are assumed to be minor contributors
to what remains predominantly a central power system.
If the level of DG "penetration" gets too
high, the phase drift features intended to make DG
device operation fail-safe will overwhelm the central
generator and cause the system to shut down.
For now, that limitation of 1547 is not a problem.
There is no way that DG will provide a large enough
fraction of our net electricity supply to manifest
the problem within the next few years. However, the
situation could start to change fairly quickly once
high temperature fuel cells for stationary power become
economically competitive.
Hybrid systems of high temperature fuel cells integrated
with gas turbines can achieve very high thermal efficiencies.
Moreover, they can do so in small modules of a megawatt
or less. 53% efficiency was demonstrated in 2002 in
a small prototype, and analysts working for DOE expect
that 60% to 70% will be common in the near future.
For advanced designs further down the line, their
target is 75 - 80%. These targets far exceed the thermal
efficiencies of today's most advanced GTCC central
power plants.
Manufacturing economies for hybrid fuel cell / gas
turbine systems appear to favor mass production of
relatively small modules. These would be in the range
of hundreds of kW to a few MW. There are no obvious
scale advantages from grouping large numbers of modules
into a central plant. For greatest efficiency, the
modules can and should be distributed among facilities
that can make use of their waste heat. But robustly
controlling a grid with the bulk of power coming from
thousands of such distributed units is not something
that IEEE 1547 was designed to handle.
Discretionary Loads
A less obvious limitation of IEEE 1547 is that it
addresses only positive DG resourcesthose capable
of delivering power to the grid. To efficiently cope
with the characteristics of wind and solar power resources,
it will be essential to have a standard that covers
negative DG resources as well. Negative DG resources
are otherwise known as discretionary (or dispatchable)
loads. Shutting down or throttling back a discretionary
load is just as effective for meeting peak demand
as turning on a peaking generator. Where it can be
made available, it's also likely to be a much cheaper
and more efficient way to meet peak demand.
While there is provision for dispatchable loads
in the national electricity market, it is applicable
only for utility companies and industrial energy users
large enough to play in the bulk energy trading market.
For ordinary commercial and residential users, the
only option at all similar has been dual metering.
Dual metering allows customers to pay lower rates
for power used during off-peak hours. Its main residential
use has been for water heating in homes that are not
supplied with gas. But it's tied to a fixed daily
schedule. It's useless for integrating wind power,
whose availability follows no set schedule.
Recently, control systems have been introduced in
the New York region that allow big air conditioners
in commercial buildings to be remotely "throttled
back" by the utility when power demand is critical.
In return for installing the controls, commercial
customers are offered lower power rates. The real-time
remote command capabilities of this system make it
closer to what's needed for the dynamic "use
it when it's there" strategy that wind and solar
resources favor. However the system is quite limited
in scope. It’s aimed at curtailing a relatively
small number of heavy loads at times when supply is
critically short. It isn't aimed at shifting discretionary
loads to times when excess supply happens to be available.
A discretionary load, by definition, has some latitude
in when it operates. The nature of the load determines
how much latitude. An example is the electrical hot
water heating mentioned earlier. The total energy
needed for water heating in one day is determined
by usage, and is not discretionary at the level of
the water heater. But because hot water is fairly
easy to store, there is considerable latitude as to
just when the energy is supplied.
Water pumping from wells to storage tanks is another
example. In that case, the timing latitude is even
larger than for water heating. While an insulated
hot water tank can hold a day’s worth of hot
water for a household, a cold water tank can easily
hold many days or even weeks of supply. That flexibility
makes water pumping probably the single most naturally
suited application for wind and solar power.
Many electrical loads that are not currently discretionary
could be made so, given incentive. For example, the
microturbine systems that Capstone Turbine Corporation
sells for small-scale CHP are normally operated to
satisfy real-time heat demand, with electricity produced
as a byproduct. However, they could easily deliver
heat to tanks of eutectic salts, which would store
it for later use. That would allow the turbines to
operate mostly at peak demand times, when their power
could command a premium.
Unfortunately, the fixed rate system that applies
for most residential and commercial customers gives
no incentive for such operation. Utilities mostly
sell power at less than their own marginal purchase
cost during peak hours, recouping by selling above
the marginal purchase cost at other times. That simplifies
metering and makes it easy for customers to understand
their utility bills, but it insulates the system from
an efficient source of matching supply and demand.
That source will be increasingly important as the
fraction of power from wind and solar resources increases.
Beyond IEEE 1547
What might a more advanced standard for interconnecting
distributed resources look like? It clearly must provide
for anti-islanding as robust and fail-safe as 1547,
while enabling very high penetration by distributed
resources. Ideally, it should make it possible to
interconnect a set of distributed resources in a semi-autonomous
grid with no central generator at all. The standard
should also allow for real-time rate variability,
in order to match available supply and demand.
What's needed is functionally similar to what already
exists for central power stations connected to one
large regional grid. Power plants linked in a regional
grid are, in their own way, "distributed resources".
But the control system for regional grids involves
human operators in busy control rooms. Reports flow
in, status displays are updated, decisions are made
and disseminated to the affected parties. Clearly,
that sort of operator control is totally infeasible
for a system integrating tens of thousands of small
power sources and discretionary loads. But is fully
automated control possible, and can it match or exceed
the safety and stability of the current system?
From a purely technical perspective, the most efficient
system might resemble the following:
-
Signal generators at local distribution stations
inject a low-amplitude signal at a frequency of
perhaps a few hundred kHz on the distribution lines.
The injected signal is modulated to provide a precise
time reference for any DG resources connected to
the line.
-
DG resources monitor the injected signal and use
it to synchronize their output. Loss of this signal
causes an immediate disconnect.
-
The signal is also modulated at a low bit rate
to convey a real-time demand level. A low demand
level encourages discretionary loads to connect
and discourages DG sources from connecting. A high
demand level does the opposite. Metering rates might
or might not be directly tied to the demand level.
- DG resources also monitor the current flowing
through the distribution line at their point of
connection. This serves two functions:
1. It allows them to sense the direction of
power flow and to perform automatic power factor
correction; and
2. It allows distributed generators to detect
and respond instantly to line faults.
The most critical feature of this scheme is the
real time demand level and how it is managed. If not
done properly, it could result in disastrous instability.
In response to a signal of low demand, large numbers
of discretionary loads could simultaneously connect,
momentarily overloading the system, only to all cut
out a moment later in response to a signal of high
demand. At least, that would be the natural fear of
the system operator.
The stability issue is actually not hard to deal
with. The solution involves hysteresis and random
delays in the connect / disconnect control logic of
individual resources. That causes them to respond
in a statistically predictable manner that preserves
system stability. Thus, in response to a lowering
of the signaled demand level, a few discretionary
loads with short delay times would connect, and/or
a few discretionary generators would disconnect. A
short time later, if the demand level remained low,
more units would respond. After a few minutes at a
given demand level, all the units that could be expected
to respond to that level would have done so. If at
that point there remained an excess of supply over
demand, the central controller, as the system's "auctioneer",
would need to further lower the signaled demand level,
triggering more units to respond.
Distributed Resources and the "Smart Grid"
Fully automated and distributed control over connection
of distributed resources as outlined above should
be technically feasible. Whether it is institutionally
feasible is another matter. It’s foreign to
the way that utilities and system operators currently
manage the power grid. It’s also foreign to
the architecture for the "smart grid" of
the future that is proposed by the Electricity Innovation
Institute in its “IntelliGrid Architecture”.
E2I, as the Electricity Innovation Institute is known,
is affiliated with the Electric Power Research Institute,
and with CEIDSConsortium for Electric Infrastructure
to support a Digital Society”. The IntelliGrid
Architecture does not envision distributed control
of individual energy resources in the manner suggested
above. It punts the issue of control for DERs by proposing
that "aggregators" manage them.
An aggregator presents much the same interface to
the system operator as a power plant. The aggregator
contracts to supply blocks of power or load in the
same manner as a power plant or a large industrial
power user. It's then the aggregator's responsibility
to somehow manage the distributed resources under
its purview so as to meet the contracted commitment.
How it achieves that is not covered by any standard,
so it’s up to individual aggregators and DER
equipment manufacturers to work out a system design.
The aggregator model is one with which incumbent
utilities and operators are comfortable; it does not
disrupt their current methods of operation to any
significant extent. Within limits, it is certainly
workable, as shown by the existence of Celerity Energy.
Celerity is the country's largest, most successful
energy aggregator, managing DE resources for the University
of California system and for many other clients. It
focuses mainly on enabling clients to get better value
out of their under-utilized backup generators. It
installs internet-connected controllers on the DE
equipment that allow it to be remotely started, stopped,
and monitored. The company maintains a database of
equipment under its management. Custom software uses
the database to negotiate contracts with the grid
operator and devise dispatch schedules for individual
pieces of equipment.
The aggregator system is able to handle discretionary
loads. In other respects, it is subject to much the
same limitations as IEEE 1547. An internet connection
is unable to provide a time reference that is sufficiently
precise for regulating phase angle, nor is it fast
enough or reliable enough to provide a trip relay.
Hence the DERs under the aggregator's control must
rely on grid sensing and a 1547-compliant grid connection
for power quality and fault response. They are therefore
subject to the same limitations on penetration level
that apply under 1547.
What’s the Alternative?
If institutional barriers prevent the adoption of
standards facilitating deployment of small DE resources,
it will likely hinder the adoption of wind and solar
energy resources. It will tend to make them more costly
and less efficient. But it won't stop them altogether.
Instead, developers of wind and solar resources will
be forced to co-develop and integrate large discretionary
loads and energy storage systems. These will allow
the integrated system to look more like conventional
power plants, as far as grid operations are concerned.
There are various ways to accomplish that economically.
One leading candidate, for example, is compressed
air energy storage (CAES). It is both a large discretionary
load, able to consume surplus power from wind or solar
resources when a surplus is available, and a very
efficient source of generation when supply is needed.
In the future, small-scale CAES may be married with
super-efficient fuel-cell / combustion turbine power
generation. That will enable maximum energy "mileage"
to be extracted from limited resources of natural
gas or gasified biomass.
Nonetheless, it would be better for the country's
energy future if appropriate standards for self-management
of small-scale DERs could be put in place. I'll
write again if I learn more about efforts in that
direction.
Readers Comments
Date |
Comment |
Roger Arnold
3.6.05 |
Wallace E. Brand sent the following comment by e-mail. I will respond to it below. - RDA
Your summary of the reasons for the change in the balance in economics
between the integrated system and distributed generation omits one very
important factor. That is the dramatic increase in cost of
transmission, distribution and substation facilities, so that
integration is more expensive. The quest for greater efficiency than
could be afforded by Edison's Big Jumbo units which had an estimated
efficiency of about 8% led to integrating over much larger areas than
the 1/2 mile radius that could be integrated with DC.
Tesla's invention of polyphase AC transmission promoted by George
Westinghouse permitted integrating larger and larger areas and
installing larger and larger central stations with increasing
efficiency and decreasing unit costs of hardware. These economies of
scale relative to small scale generation were so great they offset the
high costs of transmission and distribution. But now a single cycle
fuel cell has a greater efficiency than a single cycle turbine and a
combined cycle fuel cell or "hybrid" has a greater efficiency than a
combined cycle central station, particularly when efficiency is
measured at the customer's meter.
So the efficiency relative advantage of large scale central stations
has gone down, but at the same time the cost of the facilities of
integration needed to use them has gone up as has the cost of
electrical losses because of rising fuel costs. Part of this increase
in cost is the cost of treasure and time delay needed to combat the
increasing resistance to the wasteful use of our land resources.
The major barrier to the victory of the fuel cells distributed
generation over integrated central station service now is the lack of
sufficient sales volume to bring down fuel cell unit cost. While base
load fuel cell generation is more economic, the inability to obtain
intermediate, peaking and reserve service from the grid, is a
significant barrier to that market as the market for power is usually
one for full requirements firm power service. |
Roger Arnold
3.6.05 |
I fully agree, Wallace, that distributed generation using fuel cells
will serve to reduce load on the transmission grid, and that that will
be an economic factor in its deployment. But there are caveats.
Fuel cells require fuel, and it has to come from somewhere. Natural gas
is not looking as cheap or plentiful as once supposed. It will likely
be supplemented, and eventually replaced, by synthesis gas from
gasification of coal (and / or biomass). Coal gasification plants will
probably always be largish, centralized plants--if only because of the
logistics of transporting and handling coal and the complexities of
dealing with coal wastes. It's certainly possible to pipe the generated
gas to distributed fuel cell generators, and it's worth doing so when
there's a good use for the waste heat. But pipelines are no less
expensive than power transmission lines, in relation to the energy they
transport. Rather more so, I would think.
There's also the issue of integrating renewable energy. Large scale
wind power is always going to require long distance transmission from
the remote wind farms where it's generated to the cities where most of
it will be consumed. Solar power can often be deployed locally, but
will always require substantial energy storage capacity. The most
economical systems for energy storage--CAES and pumped hydro--tend to
be large and remote from users. So I don't expect to see transmission
lines disappearing anytime soon--unless it's to disappear underground
as part of a massive switch to HVDC power transmission. That would be
nice, now that power electronics have finally developed to the point
that HVDC can be implemented economically. Edison's revenge. |
Jeff WASHUSEN
3.8.05 |
I have just finished writing a paper for the Alternative Technology
Association in Australia explaining why it is so hard for ATA members
to get their Solar PV systems connected to the local distribution grid.
This is, primarily, a "regulatory framework" problem. Distributors are
required to provide connection to any small-scale DG owner who so
requests, but the process the DG owners have to go through is a
nightmare, and any cost advantage the small-scale DG owner creates for
the distributor and/or retailer is (typically) “confiscated” by higher
metering costs or lack of retail competition.
Basically, it looks like energy utilities are acting out their
frustrations because they lose money once the DG connects and they are
forced to deal with “nuisance” customers.
On the other hand, the regulatory framework is user-unfriendly because
regulators do not understand the technical issues, or don’t have much
empathy for small-scale GD owners. The regulators’ response is
compounded by DG owners having little understanding of the regulatory
framework, or the technical challenges that DG creates for the energy
utilities, which means the DG owners have little of the skills needed
to convince the regulators to develop a more "user-friendly" framework.
And, of course, the regulators rely on "technical" advice provided by
conservative (at best) or self-interested distributors and retailers.
This is certainly creating obstacles in Australia to wider scale
development of grid-connected DG, even though this is actively
supported by Federal Government subsidies for renewable energy through
a Photovoltaic Rebate Program.
But the issue of relevance to your article is the possibility that
penetration of small-scale DG will accelerate if Solar PV and
small-scale natural gas powered Fuel Cells become increasingly
affordable. I have noted in my report, for example, that Solar PV costs
have fallen markedly in Australia, even though the Government rebates
have been wound back. I have also noted that Origin Energy has
commenced production of its "Sliver" cell PV modules in South
Australia, which Origin claims have the potential to substantially
reduce cell costs (but not immediately); and Ballard Systems has
commenced export of Fuel Cell stacks to Ebara Ballard in Japan. Both
these technologies have real, and short-term potential to dramatically
lower unit costs for small-scale DG.
There have already been around six thousand Australian consumers
prepared to shell out AU$10,000 to AU$20,000 of their own money for a
(typically 1kW) Solar PV system at current prices; and 2,000 have
"fought" with a user-unfriendly system to get grid-connected. There may
be 10s of thousands of Australian energy users prepared to shell out
(say) $5,000 for a 1kW or 2kW. If we move from a few thousand to many
thousand grid-connected DGs, things will start to get very interesting. |
Wallace Brand
3.9.05 |
Roger: If coal gas is to be the fuel, I agree; we are back to large
scale, say 600 MW gasifiers (such as the proposed Eagle plant in Japan)
and the need for either pipelines carrying the coal gas, or
transmission lines. More likely it will be transmission lines because
the synergy of using the heat from the fuel cells can drive the
gasification process so it makes sense to located the fuel cells
adjacent to the large gasifiers.
But some think that imported natural gas from Qatar and other places
can serve for the next 20 or 30 years as natural gas will not peak for
two or three decades after the world peak of oil production. See
Deffayes, "Hubert's Peak". And the consensus is that investment in
facilities for imported natural gas can take place at a market price
for natural gas of $4 to $5. Alan Greenspan has been urging investment
and construction of such facilities but many are concerned with the
safety of them in the light of the current concern with terrorism.
But even at high priced natural gas, when hybrid fuel cells come along
with 80% efficiency, we may be back to distributed fuel cell generation
and the elimination of transmission and perhaps distribution. And even
simple cycle fuel cells can provide high efficiency when their thermal
energy can be used. A Fuel Cell Energy molten carbonate unit in Bad
Berka, Germany has been reported to have an efficiency exceeding 90%;
its thermal energy is used for both space heat and cooling. |
Roger Arnold
3.9.05 |
Regarding Jeff's posting, I'm happy to hear that Origin Energy has
started production of "sliver cell" PV modules. I've long considered
sliver cells one of the more promising technological developments on
the path to economical PV. It will take some time to bring specialized
tooling and assembly facilities on line to bring down the cost of
finished modules. But that will almost certainly happen. In the
meantime, what the technology has done is to greatly expand the output capacity that can be derived from
a given amount of silicon. That makes the finished module cost less
sensitive to the cost of silicon. It should enable the production of PV
silicon as a primary commodity--as opposed to the current situation,
where PV production is constrained by its reliance on "scrap"
electronics grade silicon.
I'm a little surprised to hear that Australia's small DG owners face
such difficult administrative and regulatory hurdles in getting grid
connected. Logically, IEEE 1547 compliance of equipment for grid
connection should be enough to address any issues the utility might
throw up--as long as the connected DG capacity on the distribution line
to the owner's site remained below a safe threshold.
One way for homeowners to avoid having to jump regulatory hurdles is
simply to install systems that don't deliver power to the grid. There's
added expense in the electronics needed to insure a one-way power flow.
In most cases, owners would also want to install some amount of storage
capacity, so that when their PV output exceeded house load, the surplus
wouldn't be wasted, and they could tap it later. On the plus side,
they'd end up with something closer to what they probably expected,
when they chose to invest in a PV system, in that they would have an
independent power source when the grid was down. (Many prospective PV
owners are dismayed to learn that, with the grid-connected systems that
are most widely installed, when the grid goes down, so does the PV
array.)
It would be ironic if the combination of regulatory hurdles, cheaper
and more efficient power electronics, and better battery technology
served to trigger a move toward one-way grid connection of PV systems.
The irony is that it would leave the utilities to face the real problem that widespread PV deployment creates for them, with no
regulatory levers available to do anything about it. The real problem
is large load swings whenever the sun disappears behind or pops out
from a good sized cloud. Utilities mostly don't have good means to deal
with large, sudden load swings, and they have been reluctant to invest
in available solutions.
Regarding Wallace's comments on NG imports, he's right, as far as the
"official forecasts" are concerned. LNG imports sufficient to maintain
gas prices at the $5 level for the next 20 - 30 years are what the EIA
is projecting. Whether the tankers and port facilities needed for that
level of imports actually get built, and whether that much gas will be
available for importing remains to be seen. Some of us are skeptical.
A quibble about the 90% efficiency quoted
for the Bad Berka CHP facility: that can't be
the thermal efficiency for electricity generation;
it's almost certainly a combined figure that
lumps electricity generated plus heat captured,
vs. heat lost in flue gases. It may be common
practice in marketing literature for CHP systems
to quote efficiency that way, but I wish they
wouldn't do it. It completely blurs the huge
difference in "exergy" (roughly, energy usable
for performing work) between electricity and
waste heat. When I wrote, in the article, that
near-term efficiencies for hybrid SOFC-CT systems
were expected to be in the 60 - 70% range, I
was talking about electrical conversion, not
about the somewhat phony CHP method of figuring
efficiency.
|
|